Electrolytics for AC coupling
2023-03-29 electronics design
There are some special considerations relevant to electrolytic capacitors in AC coupling applications, and that's the topic of this second part of the series on AC and DC coupling. Low impedances and low cutoff frequencies require high capacitance values, and electrolytic capacitors may be the only practical way, or at least a very appealing way, to achieve those values.
Throughout this discussion I'm focusing on aluminum electrolytic capacitors, which are by far the most common in audio use. When most people say "electrolytic capacitor" they mean the aluminum kind. Tantalum electrolytic capacitors also exist. They're not popular in audio, and they are different in some ways from aluminum electrolytics, but many of the same considerations also apply to them.
The big advantages of aluminum electrolytics are that they pack a lot of capacitance into a small physical space; and they are relatively cheap. The big disadvantages are that electrolytics are usually polarized; they may possibly be less reliable than other capacitors; and they may have poor specifications on electrical parameters other than raw capacitance, such as leakage, tolerance, parasitic resistance and inductance, and distortion.
I prefer to avoid electrolytics if possible anywhere they will carry audio, including for interstage coupling. I normally only use them for power supply filtering. But the high capacitance is a compelling advantage. If it's necessary to AC-couple low frequencies at low impedance, there may not really be any better choice. Then the question is how to get the best results from the electrolytic caps.
The biggest single issue in using electrolytic caps for AC coupling is that they are polarized. You're only supposed to put voltage across the cap in one direction. If the DC you are blocking happens to be in that direction, it should be fine; but if not, then maybe something bad happens. Part of the fun is that in AC coupling applications we may not know the direction of the DC in advance. Another part of the fun is that most people do not have any really clear idea of what "maybe something bad happens" means in this context.
Once in a while I've heard people ask whether it's safe to substitute an unpolarized capacitor for a polarized one. Maybe you downloaded a schematic from the Web that calls for a polarized electrolytic cap, but you have a non-polarized capacitor of some sort in the same value (maybe a special non-polarized electrolytic, maybe a big film or ceramic capacitor) and you want to use the non-polarized capacitor instead. Is that safe? In general, yes. Of course you should check the voltage ratings and so on, and there can be gotchas involving stuff like the DC-voltage derating of some very high capacitance ceramic devices in particular, but polarization as such is basically always a bad thing in capacitors, and electrolytic capacitors are usually the worst kind of capacitors. If you can substitute something non-polarized, great! You're upgrading the circuit. The trouble is, in many cases the only practical way to get the desired capacitance value at a reasonable price is with an electrolytic, and then you may be forced to use a polarized one.
I've heard a number of different stories about what it actually means for electrolytic capacitors to be polarized. A lot of people on Web fora are sure they know, and are eager to tell us, but their stories conflict. Even doing one's own experiments to test different accounts of capacitor polarization may not give a conclusive answer. If abusing a capacitor with reverse voltage might increase its failure rate from one chance in 10,000 after ten years, to one chance in 100 after one year, then I for one don't want to wait long enough and test enough capacitors to verify it myself; but that's still a pretty drastic effect from the abuse, which I'd want to avoid in my own designs.
The most convincing explanation I've found is in a series of articles by Cyril Bateman called "Capacitor Sound," which were originally published in Electronics World magazine in 2002 and 2003, and are now posted with some additional material on the Linear Audio site. The Aluminum Electrolytic Capacitor Application Guide from the Cornell Dubilier company describes very similar limits on reverse-voltage behaviour, although with a less detailed explanation of how the limits come about.
As Bateman describes it in chapter 5 of his series, an aluminum electrolytic capacitor is best understood as two capacitors in series, each created by an oxide film on a sheet of aluminum foil. The "positive" terminal of the component is connected to the anode foil. There's a relatively thick oxide film between that and the semi-liquid electrolyte. Then on the other side of the electrolyte there's a very much thinner layer of oxide between the electrolyte and the cathode foil, which connects to the "negative" terminal.
Because of the way the electrochemistry works between the electrolyte and the foil, current can flow through the oxide layers, bypassing the capacitance of the oxide, but normally only in one direction. It's as if there were a diode across the oxide layer, pointing from the electrolyte into the aluminum. Like other diodes, these ones can exhibit reverse breakdown; and the breakdown voltage depends on the thickness of the oxide layer, thus being much higher for the anode foil than the cathode foil.
Thin films have more capacitance than thick films, so the capacitance of the cathode foil is much greater than that of the anode foil. With these two capacitances in series, the overall total capacitance of the component ends up being basically equal to the smaller capacitance of the anode foil alone. (Compare to putting a high-value resistor in parallel across a lower-value resistor.)
Here's a simplified equivalent circuit diagram illustrating the effects I'm interested in. It is based on a more detailed equivalent circuit (modelling more effects) in Bateman's article.
This equivalent circuit suggests that it may be okay to apply a little bit of reverse voltage to an electrolytic capacitor, like a couple of volts, because in that state D1 is forward-biased and D2 is not breaking down. But beyond that point, with larger reverse voltages, D2 is going into breakdown mode and some significant current may flow, limited by equivalent resistances not shown in this diagram. Whether the reverse current is damaging or just annoying, and how quickly it might be damaging, isn't obvious; but at least it isn't how the capacitor is meant to operate. We probably shouldn't put more than about 1.5V of reverse voltage across the capacitor. On the other hand, we probably can safely apply that much.
Note that the limit of 1.5V is determined by the thin oxide layer on the cathode foil, which is what Bateman calls "unformed" oxide. It is not directly related to the right-direction voltage rating determined by the other foil, and using a higher-voltage electrolytic capacitor would not necessarily increase the reverse-voltage limit. Nonetheless, as Bateman also describes, capacitor manufacturers for various reasons sometimes use "formed" oxide with a higher voltage limit on the cathode too, so it is possible, not guaranteed, that some polarized aluminum electrolytic caps could actually withstand significantly more than 1.5V in the reverse direction. Capacitors marketed as non-polarized are made with the same thick-oxide "anode foil" on both sides, so they can withstand the same relatively high voltage in either direction.
There are a number of ways people deal with the polarization issue when using electrolytic caps for AC coupling, and now we may have the tools for evaluating them. Here's a summary of the most common strategies.
- Just ignore the issue and use polarized caps in what may be the wrong direction.
- Examine the DC levels on either side and attempt to make sure that the voltage difference will always be in the correct direction.
- Choose polarized caps with a much higher voltage rating than the voltages actually used in the circuit, and hope that that will mean they can also handle the actually-occurring voltages in the wrong direction as well.
- Use two polarized electrolytic capacitors back to back, with or without some kind of DC bias applied to the centre, or parallel diodes that attempt to limit reverse voltage.
- Use special non-polarized electrolytic capacitors.
Just ignoring polarization is a pretty common practice at least in DIY circles, and I have seen it in some commercial designs. If the DC voltage being blocked is small enough (up to about 1.5V, as described above) there may be no problem; similarly, if it turns out to be in the right direction by chance. In some cases where you'd use an electrolytic capacitor for AC coupling, the expected DC voltage across it is actually zero, and then (bearing in mind you would only be using these caps in low-impedance situations anyway) it's quite possible the instantaneous voltage appearing across the cap will never be more than a volt. Nonetheless, I can't recommend really just ignoring polarization.
The next step up is to be sure you know the direction of the DC voltage you are blocking. That's fine if it works. One example where it often works is when you have an op amp circuit powered from a single supply, and connections to the outside world use a 0V reference. If your internal circuitry is all running at a 4.5V reference level (as is typical in a guitar pedal) and then you are blocking that DC when you interface to the outside world at 0V, then you can be sure your blocking capacitors are blocking 4.5V DC in a specific direction. Make sure the "plus" side of each capacitor is pointing at the more-positive internal circuitry, and you should be good to go. But this kind of analysis only works in some circuits, and as discussed later, it's possible you might still want to opt for non-polarized electrolytic capacitors to reduce distortion even if you can rule out mispolarizing the regular kind.
The idea of choosing capacitors with a much higher voltage rating than you think you need, so that they will withstand the voltage you need even when it's in the wrong direction, seems to derive from the theory that the reverse voltage capability of a capacitor scales with its right-direction working voltage. If the rated forward voltage is 24V, the reasoning goes, then maybe 8V in the reverse direction is safe, and if you need to apply more reverse voltage then maybe you upgrade to a capacitor rated for 36V or 48V forward. Unfortunately, that theory is probably wrong!
If we believe Bateman - and I am inclined to, not having seen any more convincing explanations and not being so motivated as to conduct my own experiments - then the safe reverse limit is about 1.5V for polarized electrolytic capacitors in general, regardless of the forward limit, and using higher-voltage capacitors won't help much. Exceptional capacitors that handle more reverse voltage may exist but we can't depend on ours being exceptional without a specification to that effect from the manufacturer.
Using two polarized capacitors back to back is a common trick. The idea is that regardless of the direction of the applied voltage, it will be right for one of the two capacitors, and then that capacitor will drop almost all of the voltage, leaving very little to be applied in the wrong direction against the other capacitor.
The theory behind back-to-back capacitors does seem to be sound. One small gotcha is that both capacitors need to be twice the target value, because putting two of them in series cuts the value in half. To get the effect of one 100µF non-polarized capacitor we need two 200µF capacitors in series. But non-polarized electrolytics are expensive enough that two polarized units of twice the value may actually still be cheaper.
It's easy to think of ways one might try to bias or bypass the centre connection of the series-capacitor configuration to try to force the voltages into the right direction. Such an effort might include adding a high-value resistor to a power supply line, or parallel diodes across the capacitors. I've read a few discussions of these issues in various Web fora, Stack Overflow, and so on, and the general consensus seems to be that these bias and protection efforts are a bad idea. It's hard to do it right; if done badly it may end up actually increasing the reverse voltage; and it just seems to be unnecessary. The theory implies that back-to-back capacitors will protect each other in normal operation anyway. I also note that although he does not discuss this specific issue in detail, one of the cases Bateman used in his distortion measurements was the back-to-back polarized electrolytic configuration, and he did not mention there being any need to bias or protect the capacitors when doing that.
That brings us to the last strategy on the list, which is the use of special "non-polarized" electrolytic capacitors. These are made with anode foil (relatively thick oxide) on both sides, so they have a high breakdown voltage in both directions. As well as the special foil, they need to be made larger, because instead of having a very high capacitance on the cathode, they have the lower anode capacitance on both sides, effectively in series. Just as with two entire capacitors in series, having two anode foils in series means each anode foil needs twice the capacitance it would otherwise have, for the overall combination to hit the target value.
Non-polarized electrolytics are more expensive both because of being larger and because of being a specialty item made in smaller quantities. But they can withstand their full rated voltage in either direction, and a non-polarized capacitor probably still takes less board space than the equivalent series pair of polarized capacitors. Which option is cheaper overall, may vary. Also, according to Bateman's tests, the distortion introduced by a non-polarized aluminum electrolytic capacitor is significantly less than that of a pair of polarized capacitors in series. If audio quality is a high priority and you must use electrolytics, lower distortion may be a reason to use non-polarized electrolytics.
In some of his tests, Bateman actually went even further, to series pairs of non-polarized electrolytics, and found those gave even better distortion performance than single non-polarized electrolytics. As with polarized capacitors, putting them in series halves their values, so each capacitor in the pair needs to be twice the desired overall value. I think the underlying mechanism for lower distortion here is that the distortion comes from the applied voltage, and splitting the voltage across two capacitors reduces the voltage on each. But doing this means replacing an already-expensive single non-polarized capacitor with two more twice the size, and I'm not sure how practical that really is. If you are splurging on capacitors to maximize audio quality, you might be better off investing in film capacitors rather than fancy electrolytics.
◀ PREV AC and DC coupling || More about DC coupling NEXT ▶
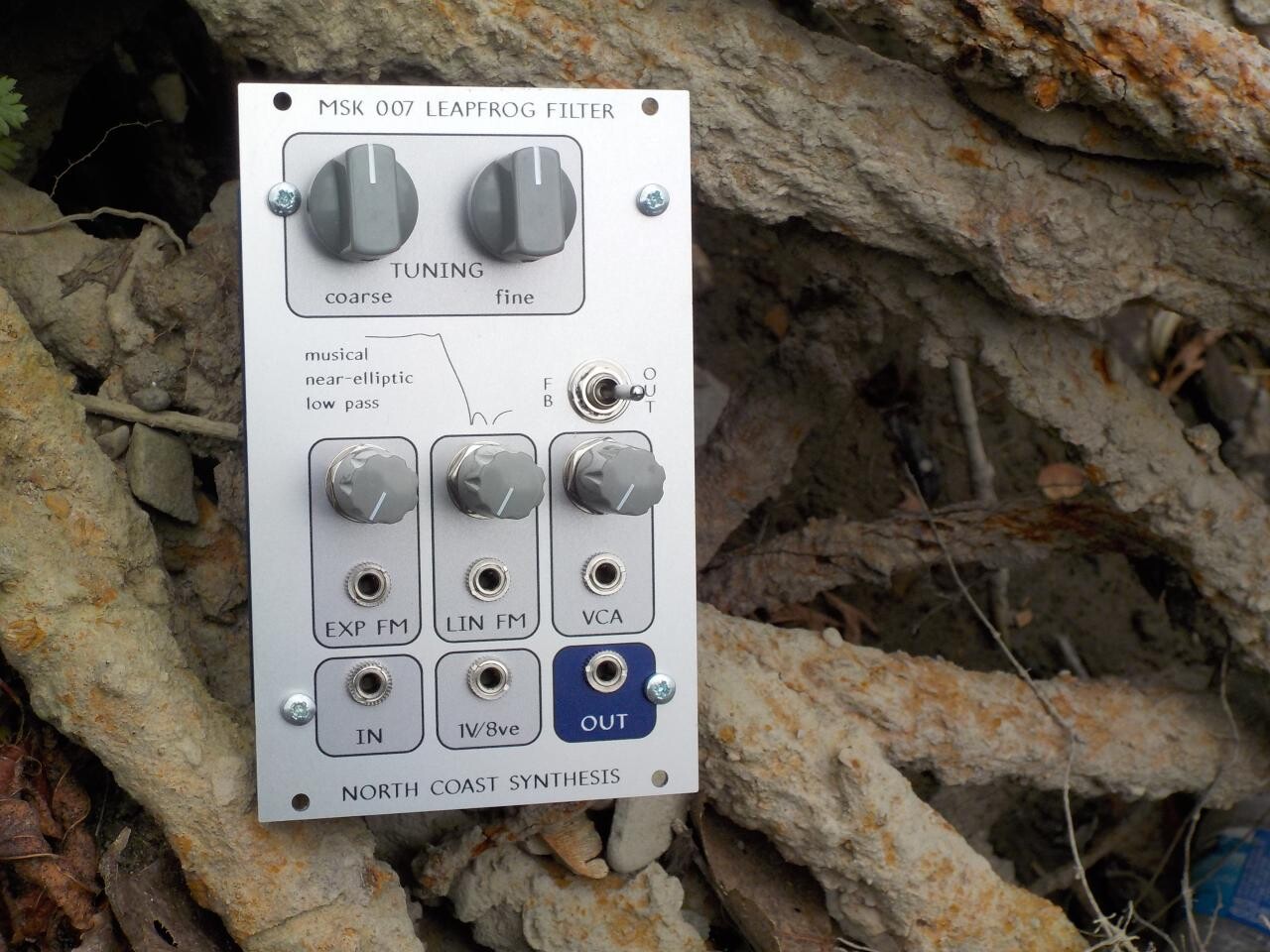